Ebola
Virus Vaccine Development
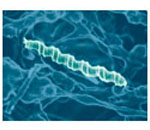 |
Tom
Foster |
Introduction
Ebola virus
is a member of the Filoviridae family, a group of enveloped,
nonsegmented, negative-strand viruses (1). Ebola first
appeared in the Democratic Republic of the Congo (formerly
Zaire) in 1976. Since that time four distinct subtypes
have been identified: Zaire, Sudan, Ivory Coast and Reston.
The virus causes severe haemorrhagic fever in human and
non-human primates, with fatality rates of 50-90% (2).
The Reston subtype is fatal to non-human primates only.
The cause of death in all subtypes is usually low blood
pressure and generalised organ failure (3), due to vascular
endothelial damage. The rapid viral replication and disease
progression affords the immune system little time to mount
a response (4). The natural reservoir of the virus remains
unknown.
The high mortality
rate, lack of any vaccine or therapy and fear over its
potential use as a biological weapon has seen a vast amount
of research undertaken into Ebola. Hence, furthering our
understanding of Ebola’s mechanisms of pathogenesis and
the development of a prophylactic vaccine are exciting
areas of immunological research.
Viral determinants and pathogenic effects
The negative-stranded
genome of the Ebola virus encodes seven structural and
regulatory proteins (5). The structural proteins consist
of a transmembrane glycoprotein (GP), a nucleoprotein
(NP), virion structural proteins (VP24, VP30, VP35, and
VP40), and a RNA-dependent RNA polymerase (6). The GP
mRNA can lead to two different glycoproteins: a transmembrane
GP and a secretory GP. Transmembrane GP is translated
after mRNA post-transcriptional modification, whereas
secretory GP (sGP) is encoded by the unedited transcript.
Research undertaken
by Yang et al (5) revealed the transmembrane GP to be
the main viral determinant in a vascular setting (7).
Experiments conducted on vein and vascular explants indicated
that vascular integrity was compromised due to expression
of GP in vivo. Through the use of deletion mutants they
identified a serine-threonine-rich, mucin-like region
responsible for GP-mediated cytotoxicity. This cytotoxicity
was maintained even after the addition of six other Ebola
gene products, indicating these genes did not contribute
to the GP toxicity. Notably these additional transfection
studies did not include treatment with VP35, for unknown
reasons. GP cytotoxicity was found to be the result of
intracellular synthesis and/or transport of the gene product
to the cell surface, since its activity was blocked by
cycloheximide (an inhibitor of protein synthesis).
Vaccine
development
Current commercial
development of an Ebola vaccine is based on the research
of the Vaccine Research Centre (VRC) within the US National
Institute of Allergy and Infectious Diseases (NIAID).
The lab, run by Nancy J. Sullivan, developed the current
Ebola prime-boost immunisation strategy.
Sullivan et
al published the first paper concerning the successful
use of an Ebola vaccine in primates in 2000 (11). A “prime-boost”
strategy was employed, whereby DNA immunisation was followed
by boosting with recombinant adenoviral (ADV) vectors
encoding pathogenic proteins. The approach relies on the
ADV boost to expand the primary T-cell response induced
by DNA vaccination (12) and generated cellular and humoral
immunity in cynomolgus macaques. Like the majority of
research discussed thus far, this study was carried out
using the Zaire subtype of the virus. The authors acknowledge
that for a vaccine to be broadly effective it would need
to provide protection against all other subtypes. The
macaques therefore received three injections of naked
DNA vectors containing both NP and three subtypes of GP
(Zaire, Ivory Coast and Sudan), at four-week intervals.
Following three months rest they were boosted with a recombinant
adenoviral vector encoding Zaire GP, before being challenged
with six plaque-forming units (PFUs) of live virus a further
three months later. All vaccinated animals survived this
lethal challenge (n=4). In vitro lymphocyte-proliferation
assays showed CD4 T cells to contribute to the cellular
immune response induced by the vaccine. This study suggests
T cell mediated and humoral immunity contribute to clearance
of the virus in non-human primates and is consistent with
previous rodent studies. However, the durability of this
response remains unknown.
Despite their
above success, the group realised the limitation of a
vaccination schedule that takes in excess of six months
to complete, particularly in the event of an acute epidemic.
The results of further non-human primate studies using
an accelerated vaccination schedule were published in
2003 (12). The accelerated schedule utilised either adenoviral
priming and boosting or simply adenoviral priming, without
the use of plasmid DNA. Cynomolgus macaques were immunised
with both ADV-GP and ADV-NP. One group were then boosted
with the same ADV vectors 9 weeks later, followed by challenge
with either a low (13 PFUs, n=4) or high (1500 PFUs, n=4)
dose of Zaire subtype Ebola virus. Alternatively, challenge
occurred four weeks after immunisation, without an ADV
boost. Results showed that adenoviral priming alone produced
a quicker, albeit weaker, immune response as compared
with the heterologous prime-boost strategy. The response
generated was, however, robust enough to confer protection
on all challenged monkeys (n=8). Protection was again
correlated with Ebola-specific CD8 T cell and antibody
responses.
Due to their
different potencies and vaccination schedules, it is envisaged
the two vaccines would serve different roles in the fight
against Ebola. The robust immune response and short administration
time of the single-shot vaccine make it particularly useful
in the event of an acute outbreak. However, the greater
potency of the prime-boost regime makes it a more effective
preventative vaccine where adequate time is available
to complete the immunisation schedule.
In November
2003 NIAID began clinical trials of the DNA component
of the prime-boost vaccination strategy. Twenty-one volunteers
received injections of the experimental vaccine to assess
its safety in humans and look for signs of any immune
response to the vaccine. Results are yet to be published.
Vical Inc., a US biotechnology company, was contracted
to manufacture the DNA vaccine and holds the right to
commercialise this component if it is successfully developed
and approved by the FDA.
Meanwhile a
Dutch company, Crucell, recently signed a US$27.6 million
contract with the NIAID to manufacture ten batches of
the recombinant adenoviral component of the vaccine (13).
In the wake of further animal studies confirming the results
of Sullivan et al this is to be used in Phase I and Phase
II clinical trials to assess the safety and potency of
the single-shot vaccine in humans.
The development
of an Ebola vaccine has been the subject of some criticism.
The efficacy of an Ebola vaccine can never be fully assessed
in large-scale human trials, due to the nature of the
virus and the need for bio-safety Level 4 containment
(14). In 2002 the FDA lowered the approval requirements
for drugs and vaccines that treat diseases caused by potential
bio-weapons, where human efficacy trials would be impossible
or unethical. Under the alternative approval process preclinical
data showing efficacy in two relevant animal models, combined
with Phase I study results is sufficient to permit approval.
Also as part of the US governments “Project BioShield”,
which President Bush signed into law in July 2004, US$5.6
billion has been allocated over ten years to purchase
next generation countermeasures against chemical, biological,
radiological or nuclear agents. This effectively creates
a market for a product such as the Ebola vaccine that
would otherwise not exist.
While the results
of Sullivan et al have been widely applauded, their significance
has been questioned in light of the relatively small amount
(6 PFU) of virus used in their initial experiments (15).
This was to some extent rectified in their single-dose
vaccine studies where the highest does individual macaques
were challenged with was 1762 PFUs.
The high incidence
of adenoviral antibodies in the global population has
been raised as a potential weakness of any strategy that
utilises adenoviral vectors to stimulate a response. This
is due to the potential for an immune response to be mounted
against the vector itself. This has been addressed by
using a rare serotype of adenovirus 35 (16). However like
most concerns over the Ebola vaccine, a definitive resolution
requires further deadly outbreaks of the virus.
A number of
interesting ethical questions have also been raised regarding
the disproportionate amount of money being spent on developing
a vaccine for a virus that has killed less than two thousand
people. The argument goes that perhaps the money would
be better-spent developing treatments that would better
serve the wider population. In the context of western
Africa, the impact of malaria and AIDS treatments, for
example, would be significantly greater.
Non-human Primates and the Natural Reservoir
Recent epidemiological
investigations have been carried out in the wake of a
series of Ebola Zaire outbreaks in western central Africa
in 2001-2002 (17). By sequencing the entire open reading
frame of Ebola GP, these outbreaks were shown to be from
multiple introductions of the virus from different infected
animal sources. The observed high incidence of the virus
amongst the local population of hunters was confirmed.
All index cases were infected when handling dead animals.
The implicated animals include gorilla, chimpanzee, and
duiker. It is thought that humans and duikers scavenging
for meat probably became infected by contact with dead
apes. Interestingly, tissue samples indicate these animals
died without developing specific IgG responses to the
virus.
Outbreaks of
Ebola in non-human primates have been known to precede
human infection. This was the proven to be the case in
these recent outbreaks. The ramification for West Africa’s
gorilla population is therefore significant. Eight groups
of gorillas, totally 143 individuals, disappeared and
have not been seen since the last outbreak of Ebola in
the area. These gorillas had been monitored almost daily
for ten years. The rapid, localised spread of the virus
amongst such populations combined with their slow reproductive
cycles, hunting and poaching may lead to their extinction
in western Africa.
The natural
reservoir for Ebola remains unknown. Due to the susceptibility
of non-human primates to the virus, they are not the natural
reservoir (18). Screening of thousands of vertebrates
and invertebrates have found only bats able to support
the replication and circulation of high titres of virus,
without developing disease (18). This has lead to the
hypothesis that these mammals may play a role in maintaining
the virus in the tropical forest (2). Further conclusive
evidence is required.
Conclusion
The development
of an Ebola vaccine, and the research surrounding it,
are undoubtedly fascinating areas of immunological research.
To date, most research has focused on the protective efficacies
of immune responses to viral GP and NP. The promise such
an approach holds has been confirmed in non-human primate
models. Combined with the current political environment,
this has lead to the establishment of commercial relationships
between industry and US research institutions for the
manufacture and further testing of two vaccine types.
The heterologous prime-boost and single shot adenoviral
vaccines differ in their administration schedule, vaccine
components, potency of induced immune response and therefore
their intended use. The development of an Ebola vaccine
also raises some significant moral questions, given its
low incidence. Unfortunately definitive answers regarding
the efficacy of developed vaccines will not be known until
further outbreaks of the virus occur.
References
Rao, M., M.
Bray, C. R. Alving, P. Jahrling, G. R. Matyas. 2002. Induction
of Immune Responses in Mice and Monkeys to Ebola Virus
after Immunisation with Liposome-Encapsulated Irradiated
Ebola Virus: Protection in Mice Requires CD4+ T Cells.
Journal of Virology. 76(18):9176
World Health
Organisation.
Fact sheet No.103: Ebola haemorrhagic fever. Accessed
10 May 2005
Crucell. Fact
sheet: About Ebola. Accessed 7 May 2005
Nabel, G.J.
2003. Vaccine for AIDS and Ebola virus infection. Virus
Research. 92:213
Yang, Z. Y.,
R. Delgado, L. Xu, R. F. Todd, E. G. Nabel, A. Sanchez,
and G. J. Nabel. 1998. Distinct Cellular Interactions
of Secreted and Transmembrane Ebola Virus Glycoproteins.
Science. 279:1034
Wilson, J.
A., M. Bray, R. Bakken, and M. K. Hart. 2001. Vaccine
potential of Ebola Virus VP24, VP30, VP35, and VP40 Proteins.
Virology. 286:384
Yang, Z. Y.,
H. J. Duckers, N. J. Sullivan, A. Sanchez, E. G. Nabel,
and G. J. Nabel. 2000. Identification of the Ebola virus
glycoprotein as the main viral determinant of vascular
cell cytotoxicity and injury. Nature. 6(8):886
. Sullivan, N. J., A. Sanchex, P. E. Rollin, Z. Y. Yang,
and G. J. Nabel. 2000. Development of a preventative vaccine
for Ebola virus infection in primates. Nature. 408:605
Sullivan,
N. J., T. W. Geisbert, J. B. Geisbert, L. Xu, Z. Y. Yang,
M. Roederer, T. A. Koup, P. B. Jahrling, G. J. Nabel.
2003. Accelerated vaccination for Ebola virus haemorrhagic
fever in non-human primates. Nature. 424:681
Crucell. Press
release. Accessed 14 May 2005
Gibbs, W.
W. 2004. An uncertain defence. Scientific American. 291(4):20
Burton, D.
R., and P. W. H. I. Parren. 2000. Fighting the Ebola virus.
Nature. 408:527
Dove, A. 2002.
Ebola vaccine gets corporate backer. Nature Medicine 8(7):645
Leroy, E.
M., P. Rouquet, P. Formenty, S. Souquiere, A. Kilbourne,
J. M. Froment, M. Bermejo, S. Smit, W. Karesh, R. Swanepoel,
S. R. Zaki, and P. E. Rollin. 2004. Mutiple Ebola Virus
Transmission Events and Rapid Decline of Central African
Wildlife. Science. 303:387
Colebuners,
R., and M. Borchet. 2000. Ebola Haemorrhagic Fever – a
Review. Journal of Infection. 40:16
|